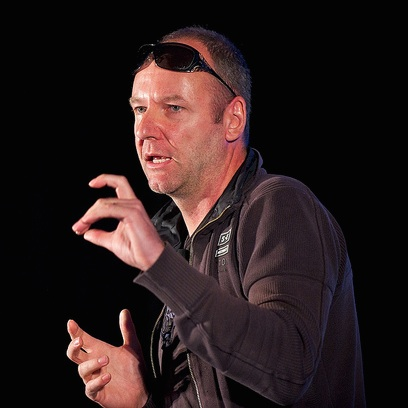
Hartmut Neven
Hartmut Neven is an Vice President of Engineering at Google. He is the founder and manager of the Quantum Artificial Intelligence lab. The objective of the lab is to fabricate quantum processors and develop novel quantum algorithms to dramatically accelerate computational tasks for machine intelligence. Previously, Hartmut was head of the Visual Search team. His team developed the visual search service which today is used by a large number of Google products including Image Search, Google Photos, YouTube, Street View and Google Goggles. His teams won a number of competitions designed to establish the best visual recognition software for faces (FERET 1996, FRVT 2002), objects (ImageNet 2014) and text (ICDAR 2013). Hartmut was also a co-founder of project Glass and led the team that built the first prototype. Prior to joining Google, Hartmut started two computer vision companies, the second one was acquired by Google in 2006. Hartmut obtained his Ph.D. in 1996 with a thesis on "Dynamics for vision-guided autonomous mobile robots". Then he became a research professor for computer science and theoretical neuroscience at the University of Southern California.
Authored Publications
Sort By
Google
Visualizing Dynamics of Charges and Strings in (2+1)D Lattice Gauge Theories
Tyler Cochran
Bernhard Jobst
Yuri Lensky
Gaurav Gyawali
Norhan Eassa
Melissa Will
Aaron Szasz
Dmitry Abanin
Rajeev Acharya
Laleh Beni
Trond Andersen
Markus Ansmann
Frank Arute
Kunal Arya
Abe Asfaw
Juan Atalaya
Brian Ballard
Alexandre Bourassa
Michael Broughton
David Browne
Brett Buchea
Bob Buckley
Tim Burger
Nicholas Bushnell
Anthony Cabrera
Juan Campero
Hung-Shen Chang
Jimmy Chen
Benjamin Chiaro
Jahan Claes
Agnetta Cleland
Josh Cogan
Roberto Collins
Paul Conner
William Courtney
Alex Crook
Ben Curtin
Sayan Das
Laura De Lorenzo
Agustin Di Paolo
Paul Donohoe
ILYA Drozdov
Andrew Dunsworth
Alec Eickbusch
Aviv Elbag
Mahmoud Elzouka
Vinicius Ferreira
Ebrahim Forati
Austin Fowler
Brooks Foxen
Suhas Ganjam
Robert Gasca
Élie Genois
William Giang
Dar Gilboa
Raja Gosula
Alejo Grajales Dau
Dietrich Graumann
Alex Greene
Steve Habegger
Monica Hansen
Sean Harrington
Paula Heu
Oscar Higgott
Jeremy Hilton
Robert Huang
Ashley Huff
Bill Huggins
Cody Jones
Chaitali Joshi
Pavol Juhas
Hui Kang
Amir Karamlou
Kostyantyn Kechedzhi
Trupti Khaire
Bryce Kobrin
Alexander Korotkov
Fedor Kostritsa
John Mark Kreikebaum
Vlad Kurilovich
Dave Landhuis
Tiano Lange-Dei
Brandon Langley
Kim Ming Lau
Justin Ledford
Kenny Lee
Loick Le Guevel
Wing Li
Alexander Lill
Will Livingston
Daniel Lundahl
Aaron Lunt
Sid Madhuk
Ashley Maloney
Salvatore Mandra
Leigh Martin
Orion Martin
Cameron Maxfield
Seneca Meeks
Anthony Megrant
Reza Molavi
Sebastian Molina
Shirin Montazeri
Ramis Movassagh
Charles Neill
Michael Newman
Murray Ich Nguyen
Chia Ni
Kris Ottosson
Alex Pizzuto
Rebecca Potter
Orion Pritchard
Ganesh Ramachandran
Matt Reagor
David Rhodes
Gabrielle Roberts
Kannan Sankaragomathi
Henry Schurkus
Mike Shearn
Aaron Shorter
Noah Shutty
Vladimir Shvarts
Vlad Sivak
Spencer Small
Clarke Smith
Sofia Springer
George Sterling
Jordan Suchard
Alex Sztein
Doug Thor
Mert Torunbalci
Abeer Vaishnav
Justin Vargas
Sergey Vdovichev
Guifre Vidal
Steven Waltman
Shannon Wang
Brayden Ware
Kristi Wong
Cheng Xing
Jamie Yao
Ping Yeh
Bicheng Ying
Juhwan Yoo
Grayson Young
Yaxing Zhang
Ningfeng Zhu
Yu Chen
Vadim Smelyanskiy
Adam Gammon-Smith
Frank Pollmann
Michael Knap
Nature, 642 (2025), 315–320
Quantum Computation of Stopping power for Inertial Fusion Target Design
Dominic Berry
Alina Kononov
Alec White
Joonho Lee
Andrew Baczewski
Proceedings of the National Academy of Sciences, 121 (2024), e2317772121
Dynamics of magnetization at infinite temperature in a Heisenberg spin chain
Trond Andersen
Rhine Samajdar
Andre Petukhov
Jesse Hoke
Dmitry Abanin
ILYA Drozdov
Xiao Mi
Alexis Morvan
Charles Neill
Rajeev Acharya
Richard Ross Allen
Kyle Anderson
Markus Ansmann
Frank Arute
Kunal Arya
Abe Asfaw
Juan Atalaya
Gina Bortoli
Alexandre Bourassa
Leon Brill
Michael Broughton
Bob Buckley
Tim Burger
Nicholas Bushnell
Juan Campero
Hung-Shen Chang
Jimmy Chen
Benjamin Chiaro
Desmond Chik
Josh Cogan
Roberto Collins
Paul Conner
William Courtney
Alex Crook
Ben Curtin
Agustin Di Paolo
Andrew Dunsworth
Clint Earle
Lara Faoro
Edward Farhi
Reza Fatemi
Vinicius Ferreira
Ebrahim Forati
Austin Fowler
Brooks Foxen
Gonzalo Garcia
Élie Genois
William Giang
Dar Gilboa
Raja Gosula
Alejo Grajales Dau
Steve Habegger
Michael Hamilton
Monica Hansen
Sean Harrington
Paula Heu
Gordon Hill
Markus Hoffmann
Trent Huang
Ashley Huff
Bill Huggins
Sergei Isakov
Justin Iveland
Cody Jones
Pavol Juhas
Marika Kieferova
Alexei Kitaev
Andrey Klots
Alexander Korotkov
Fedor Kostritsa
John Mark Kreikebaum
Dave Landhuis
Pavel Laptev
Kim Ming Lau
Lily Laws
Joonho Lee
Kenny Lee
Yuri Lensky
Alexander Lill
Wayne Liu
Salvatore Mandra
Orion Martin
Steven Martin
Seneca Meeks
Amanda Mieszala
Shirin Montazeri
Ramis Movassagh
Wojtek Mruczkiewicz
Ani Nersisyan
Michael Newman
JiunHow Ng
Murray Ich Nguyen
Tom O'Brien
Seun Omonije
Alex Opremcak
Rebecca Potter
Leonid Pryadko
David Rhodes
Charles Rocque
Negar Saei
Kannan Sankaragomathi
Henry Schurkus
Christopher Schuster
Mike Shearn
Aaron Shorter
Noah Shutty
Vladimir Shvarts
Vlad Sivak
Jindra Skruzny
Clarke Smith
Rolando Somma
George Sterling
Doug Strain
Marco Szalay
Doug Thor
Alfredo Torres
Guifre Vidal
Cheng Xing
Jamie Yao
Ping Yeh
Juhwan Yoo
Grayson Young
Yaxing Zhang
Ningfeng Zhu
Jeremy Hilton
Anthony Megrant
Yu Chen
Vadim Smelyanskiy
Vedika Khemani
Sarang Gopalakrishnan
Tomaž Prosen
Science, 384 (2024), pp. 48-53
Stable quantum-correlated many-body states through engineered dissipation
Xiao Mi
Alexios Michailidis
Sara Shabani
Jerome Lloyd
Rajeev Acharya
Igor Aleiner
Trond Andersen
Markus Ansmann
Frank Arute
Kunal Arya
Abe Asfaw
Juan Atalaya
Gina Bortoli
Alexandre Bourassa
Leon Brill
Michael Broughton
Bob Buckley
Tim Burger
Nicholas Bushnell
Jimmy Chen
Benjamin Chiaro
Desmond Chik
Charina Chou
Josh Cogan
Roberto Collins
Paul Conner
William Courtney
Alex Crook
Ben Curtin
Alejo Grajales Dau
Dripto Debroy
Agustin Di Paolo
ILYA Drozdov
Andrew Dunsworth
Lara Faoro
Edward Farhi
Reza Fatemi
Vinicius Ferreira
Ebrahim Forati
Austin Fowler
Brooks Foxen
Élie Genois
William Giang
Dar Gilboa
Raja Gosula
Steve Habegger
Michael Hamilton
Monica Hansen
Sean Harrington
Paula Heu
Markus Hoffmann
Trent Huang
Ashley Huff
Bill Huggins
Sergei Isakov
Justin Iveland
Cody Jones
Pavol Juhas
Kostyantyn Kechedzhi
Marika Kieferova
Alexei Kitaev
Andrey Klots
Alexander Korotkov
Fedor Kostritsa
John Mark Kreikebaum
Dave Landhuis
Pavel Laptev
Kim Ming Lau
Lily Laws
Joonho Lee
Kenny Lee
Yuri Lensky
Alexander Lill
Wayne Liu
Orion Martin
Amanda Mieszala
Shirin Montazeri
Alexis Morvan
Ramis Movassagh
Wojtek Mruczkiewicz
Charles Neill
Ani Nersisyan
Michael Newman
JiunHow Ng
Murray Ich Nguyen
Tom O'Brien
Alex Opremcak
Andre Petukhov
Rebecca Potter
Leonid Pryadko
Charles Rocque
Negar Saei
Kannan Sankaragomathi
Henry Schurkus
Christopher Schuster
Mike Shearn
Aaron Shorter
Noah Shutty
Vladimir Shvarts
Jindra Skruzny
Clarke Smith
Rolando Somma
George Sterling
Doug Strain
Marco Szalay
Alfredo Torres
Guifre Vidal
Cheng Xing
Jamie Yao
Ping Yeh
Juhwan Yoo
Grayson Young
Yaxing Zhang
Ningfeng Zhu
Jeremy Hilton
Anthony Megrant
Yu Chen
Vadim Smelyanskiy
Dmitry Abanin
Science, 383 (2024), pp. 1332-1337
Optimizing quantum gates towards the scale of logical qubits
Alexandre Bourassa
Andrew Dunsworth
Will Livingston
Vlad Sivak
Trond Andersen
Yaxing Zhang
Desmond Chik
Jimmy Chen
Charles Neill
Alejo Grajales Dau
Anthony Megrant
Alexander Korotkov
Vadim Smelyanskiy
Yu Chen
Nature Communications, 15 (2024), pp. 2442
Quantum Simulation of Exact Electron Dynamics can be more Efficient than Classical Mean-Field Methods
William J. Huggins
Dominic W. Berry
Shu Fay Ung
Andrew Zhao
David Reichman
Andrew Baczewski
Joonho Lee
Nature Communications, 14 (2023), pp. 4058
Purification-Based Quantum Error Mitigation of Pair-Correlated Electron Simulations
Thomas E O'Brien
Gian-Luca R. Anselmetti
Fotios Gkritsis
Vincent Elfving
Stefano Polla
William J. Huggins
Oumarou Oumarou
Kostyantyn Kechedzhi
Dmitry Abanin
Rajeev Acharya
Igor Aleiner
Richard Ross Allen
Trond Ikdahl Andersen
Kyle Anderson
Markus Ansmann
Frank Carlton Arute
Kunal Arya
Juan Atalaya
Michael Blythe Broughton
Bob Benjamin Buckley
Alexandre Bourassa
Leon Brill
Tim Burger
Nicholas Bushnell
Jimmy Chen
Yu Chen
Benjamin Chiaro
Desmond Chun Fung Chik
Josh Godfrey Cogan
Roberto Collins
Paul Conner
William Courtney
Alex Crook
Ben Curtin
Ilya Drozdov
Andrew Dunsworth
Daniel Eppens
Lara Faoro
Edward Farhi
Reza Fatemi
Ebrahim Forati
Austin Fowler
Brooks Riley Foxen
William Giang
Dar Gilboa
Alejandro Grajales Dau
Steve Habegger
Michael C. Hamilton
Sean Harrington
Jeremy Patterson Hilton
Markus Rudolf Hoffmann
Trent Huang
Ashley Anne Huff
Sergei Isakov
Justin Thomas Iveland
Cody Jones
Pavol Juhas
Marika Kieferova
Andrey Klots
Alexander Korotkov
Fedor Kostritsa
John Mark Kreikebaum
Dave Landhuis
Pavel Laptev
Kim Ming Lau
Lily MeeKit Laws
Joonho Lee
Kenny Lee
Alexander T. Lill
Wayne Liu
Orion Martin
Trevor Johnathan Mccourt
Anthony Megrant
Xiao Mi
Masoud Mohseni
Shirin Montazeri
Alexis Morvan
Ramis Movassagh
Wojtek Mruczkiewicz
Charles Neill
Ani Nersisyan
Michael Newman
Jiun How Ng
Murray Nguyen
Alex Opremcak
Andre Gregory Petukhov
Rebecca Potter
Kannan Aryaperumal Sankaragomathi
Christopher Schuster
Mike Shearn
Aaron Shorter
Vladimir Shvarts
Jindra Skruzny
Vadim Smelyanskiy
Clarke Smith
Rolando Diego Somma
Doug Strain
Marco Szalay
Alfredo Torres
Guifre Vidal
Jamie Yao
Ping Yeh
Juhwan Yoo
Grayson Robert Young
Yaxing Zhang
Ningfeng Zhu
Christian Gogolin
Nature Physics (2023)
Measurement-induced entanglement and teleportation on a noisy quantum processor
Jesse Hoke
Matteo Ippoliti
Dmitry Abanin
Rajeev Acharya
Trond Andersen
Markus Ansmann
Frank Arute
Kunal Arya
Abe Asfaw
Juan Atalaya
Gina Bortoli
Alexandre Bourassa
Leon Brill
Michael Broughton
Bob Buckley
Tim Burger
Nicholas Bushnell
Jimmy Chen
Benjamin Chiaro
Desmond Chik
Josh Cogan
Roberto Collins
Paul Conner
William Courtney
Alex Crook
Ben Curtin
Alejo Grajales Dau
Agustin Di Paolo
ILYA Drozdov
Andrew Dunsworth
Daniel Eppens
Edward Farhi
Reza Fatemi
Vinicius Ferreira
Ebrahim Forati
Austin Fowler
Brooks Foxen
William Giang
Dar Gilboa
Raja Gosula
Steve Habegger
Michael Hamilton
Monica Hansen
Paula Heu
Markus Hoffmann
Trent Huang
Ashley Huff
Bill Huggins
Sergei Isakov
Justin Iveland
Cody Jones
Pavol Juhas
Kostyantyn Kechedzhi
Marika Kieferova
Alexei Kitaev
Andrey Klots
Alexander Korotkov
Fedor Kostritsa
John Mark Kreikebaum
Dave Landhuis
Pavel Laptev
Kim Ming Lau
Lily Laws
Joonho Lee
Kenny Lee
Yuri Lensky
Alexander Lill
Wayne Liu
Orion Martin
Amanda Mieszala
Shirin Montazeri
Alexis Morvan
Ramis Movassagh
Wojtek Mruczkiewicz
Charles Neill
Ani Nersisyan
Michael Newman
JiunHow Ng
Murray Ich Nguyen
Tom O'Brien
Seun Omonije
Alex Opremcak
Andre Petukhov
Rebecca Potter
Leonid Pryadko
Charles Rocque
Negar Saei
Kannan Sankaragomathi
Henry Schurkus
Christopher Schuster
Mike Shearn
Aaron Shorter
Noah Shutty
Vladimir Shvarts
Jindra Skruzny
Clarke Smith
Rolando Somma
George Sterling
Doug Strain
Marco Szalay
Alfredo Torres
Guifre Vidal
Cheng Xing
Jamie Yao
Ping Yeh
Juhwan Yoo
Grayson Young
Yaxing Zhang
Ningfeng Zhu
Jeremy Hilton
Anthony Megrant
Yu Chen
Vadim Smelyanskiy
Xiao Mi
Vedika Khemani
Nature, 622 (2023), 481–486
Suppressing quantum errors by scaling a surface code logical qubit
Jeremy Hilton
Jimmy Chen
Juan Atalaya
Kenny Lee
Michael Newman
Vadim Smelyanskiy
Yu Chen
Abe Asfaw
Anthony Megrant
Cody Jones
Nature (2023)
Noise-resilient Majorana Edge Modes on a Chain of Superconducting Qubits
Abe Asfaw
Alejandro Grajales Dau
Alex Crook
Alex Opremcak
Alexa Rubinov
Alexander Korotkov
Alexandre Bourassa
Alexei Kitaev
Alexis Morvan
Andre Gregory Petukhov
Andrew Dunsworth
Andrey Klots
Anthony Megrant
Ashley Anne Huff
Austin Fowler
Benjamin Chiaro
Bernardo Meurer Costa
Bob Benjamin Buckley
Brooks Foxen
Charles Neill
Christopher Schuster
Cody Jones
Daniel Eppens
Dar Gilboa
Dave Landhuis
Dmitry Abanin
Doug Strain
Ebrahim Forati
Edward Farhi
Emily Mount
Fedor Kostritsa
Frank Carlton Arute
Guifre Vidal
Igor Aleiner
Jamie Yao
Jeremy Patterson Hilton
Joao Basso
John Mark Kreikebaum
Joonho Lee
Juan Atalaya
Juhwan Yoo
Justin Thomas Iveland
Kannan Aryaperumal Sankaragomathi
Kenny Lee
Kim Ming Lau
Kostyantyn Kechedzhi
Kunal Arya
Lara Faoro
Leon Brill
Marco Szalay
Markus Rudolf Hoffmann
Masoud Mohseni
Michael Blythe Broughton
Michael Newman
Michel Henri Devoret
Mike Shearn
Nicholas Bushnell
Orion Martin
Paul Conner
Pavel Laptev
Ping Yeh
Rajeev Acharya
Rebecca Potter
Reza Fatemi
Roberto Collins
Sergei Isakov
Shirin Montazeri
Steve Habegger
Thomas E O'Brien
Trent Huang
Trond Ikdahl Andersen
Vadim Smelyanskiy
Vladimir Shvarts
Wayne Liu
William Courtney
William Giang
William J. Huggins
Wojtek Mruczkiewicz
Xiao Mi
Yaxing Zhang
Yu Chen
Yuan Su
Zijun Chen
Science (2022) (to appear)