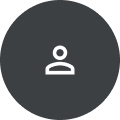
Peter H. Hochschild
Authored Publications
Sort By
Google
Cores that don't count
Rama Krishna Govindaraju
Proc. 18th Workshop on Hot Topics in Operating Systems (HotOS 2021)
Sundial: Fault-tolerant Clock Synchronization for Datacenters
Hema Hariharan
Dave Platt
Simon Sabato
Minlan Yu
Prashant Chandra
14th USENIX Symposium on Operating Systems Design and Implementation (OSDI 20), USENIX Association (2020), pp. 1171-1186
Spanner: Google's Globally Distributed Database
Preview
Michael Epstein
Andrew Fikes
Christopher Frost
J. J. Furman
Andrey Gubarev
Christopher Heiser
Sebastian Kanthak
Eugene Kogan
Hongyi Li
Sergey Melnik
David Mwaura
David Nagle
Rajesh Rao
Lindsay Rolig
Yasushi Saito
Michal Szymaniak
Christopher Taylor
Ruth Wang
Dale Woodford
ACM Trans. Comput. Syst., 31 (2013), pp. 8