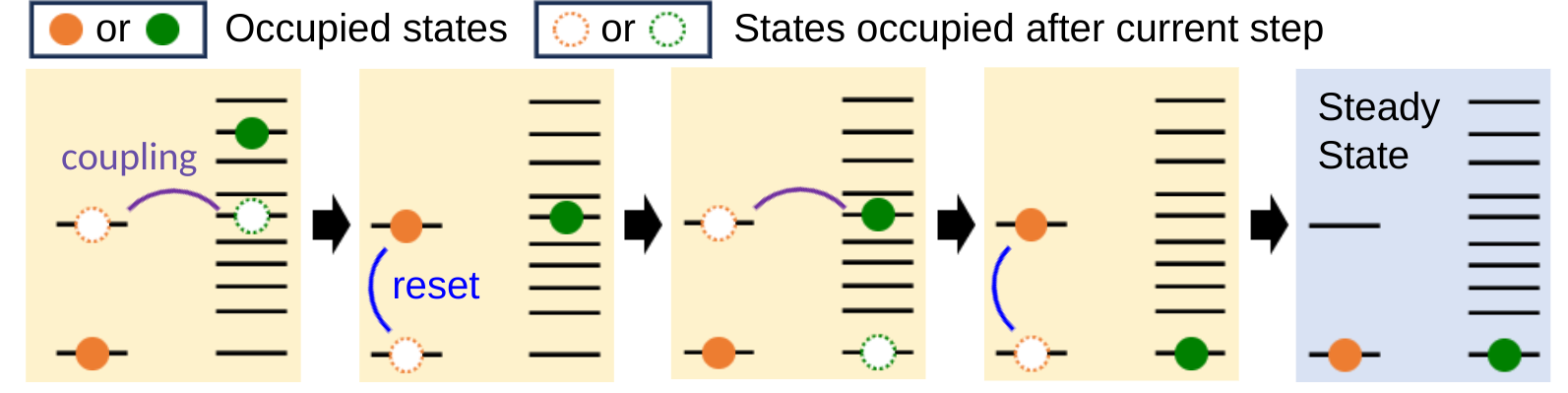
Preparing and stabilizing quantum states through engineered dissipation
April 9, 2024
Xiao Mi and Dmitry Abanin, Research Scientists, Google Research, Quantum AI Team
In our latest work, published in Science, we explore a counterintuitive effect of environmental dissipation which is often viewed as harmful to quantum computing. By carefully engineering dissipation, a new scalable protocol for preparing entangled many-body quantum states with lifetimes longer than physical qubits was discovered.
Quick links
A quantum computer can solve certain problems exponentially faster than a classical computer. However, current realizations of its building blocks, qubits, are plagued by errors induced by uncontrolled interactions between quantum bits (or qubits) and the environment. Such interactions introduce dissipation, which destroys quantum entanglement, a necessary resource in quantum algorithms, and drives a quantum processor toward trivial, classical states. This decoherence process introduces errors, corrupting the utility of the quantum processor. As such, environmental dissipation is the main limiting factor for the current-generation quantum processors. Paradoxically, while uncontrolled dissipation is a bane of current quantum processors, engineered dissipation — created with a carefully tailored environment — may in fact aid a quantum processor by steering it into a desired entangled state.
In our latest work, “Stable Quantum-Correlated Many Body States via Engineered Dissipation”, published in Science, we explore this counterintuitive effect of engineered dissipation. Not only does this method offer a reliable way to prepare a quantum state, but it also naturally stabilizes the state so that it survives much longer than it would without the dissipation. These experiments lay the groundwork for improved methods to prepare strongly correlated states (which may be found in e.g. magnets) in a quantum processor, which could help physicists learn about exotic quantum materials, such as high-temperature superconductors, in the future.
Background
Before a quantum processor can run an algorithm or simulation, all of its qubits must be initialized into a known state. This process, known as quantum state preparation, is often accomplished by applying quantum operations that are coherent — that is, they do not induce any dissipation. In this work, we instead couple the qubits to an environment that is tailored in just the right way so that the qubits are steered to their desired state through dissipation.
To demonstrate how this works, we can look at one of the simplest examples, where engineered dissipation is used to “cool” a quantum system toward its lowest energy state, known as its ground state. To accomplish this, we first connect the quantum system to another, auxiliary quantum system. Next we can transfer excitations from the system of interest to the auxiliary system, then remove the excitations via engineered dissipation. The removal of excitations gradually drives the system towards its entangled ground state (see illustration below).
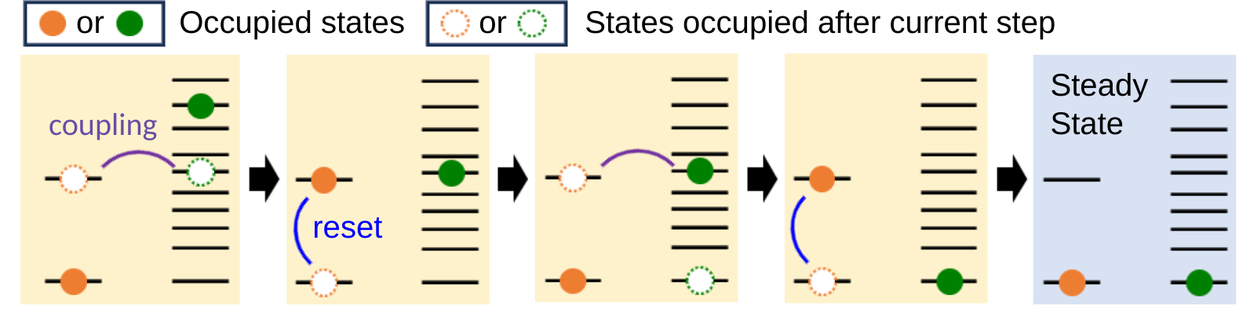
Dissipative cooling scheme. The target qubit (green) is coupled to an auxiliary qubit (orange) to transfer energy from the target to the auxiliary. Then a dissipative “reset” operation removes the energy from the auxiliary. After repeating this process many times, both the target and auxiliary are left in their ground states.
In the past, some groups have prepared entangled states of up to eight atoms with engineered dissipation. But more intricate, many-body entangled states have been out of reach due to environmental decoherence caused by other, undesirable dissipation channels. With our Sycamore processor, we have developed fast reset capabilities that makes the desired engineered dissipation dominant over unwanted dissipation channels.
Experiment
In this work, we use 35 qubits of a 49-qubit Sycamore processor to emulate magnetic spins, while the 14 remaining auxiliary qubits are used to mimic the previously mentioned tailored environment. We found that over time, this tailored environment imparts a cooling effect on the spin system and steers it toward the entangled ground state of a quantum spin model known as the transverse-field Ising model. In this model, an array of spins are positioned on a lattice, with each either pointing “spin-up” or “spin-down.” Neighboring spins interact with one another, and an external magnetic field is applied perpendicular to the spin alignment. Depending on the relative strengths between the spin interactions and the applied magnetic field, the system can be ferromagnetic or paramagnetic.
We demonstrate the effect of cooling on the spins in this model through a series of experiments. First, we set up a 1D system, where a chain of our “spin” qubits are connected in a line. Throughout this chain, every few qubits are also connected to auxiliaries that act as the dissipative environment (see figure below). We found that as the cooling cycles progress, the system settles into a correlated state that resembles the ground state of the 1D transverse-field Ising model. We then connect our qubits in a two-dimensional lattice (with auxiliaries dispersed throughout; see the middle panel below) to perform a similar experiment in 2D. We find that, in this 2D system, we can create antiferromagnetic correlations between the spins so that they anti-align with their neighbors.
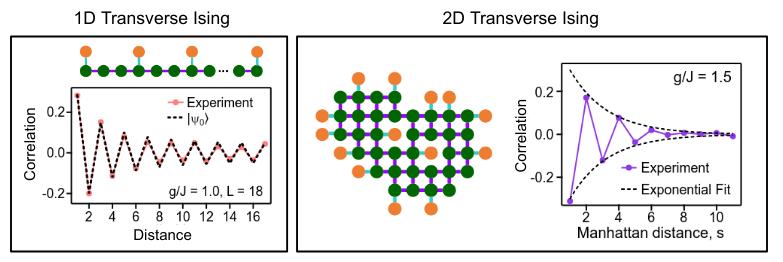
Dissipatively cooling a transverse Ising model. Left: 1D chain of up to 30 qubits (green) emulating a 1D transverse Ising model. An array of auxiliary qubits (orange) act as dissipative baths that remove excitations from the 1D chain cooling it toward ground state. After the cooling process, we observe long-ranged correlations between qubits that resemble closely the ground state of the Ising chain (|φ0⟩). Right: 2D array of 35 qubits (green) emulating a 2D transverse Ising model, cooled by 14 auxiliary qubits (orange). The final state also exhibits long range correlations, shown on the right.
When we apply techniques previously developed to mitigate errors, we found that we could dissipatively prepare ground states with fidelities of 90% for an 18-qubit system. This value matches the highest achieved fidelities at comparable system sizes using unitary circuits. We also demonstrated theoretically, in the same work, that the dissipative state preparation protocol scales more favorably vs. system size in terms of preserving state fidelity. These comparisons highlight the promise of the dissipative approach. The cooling algorithm is expected to be even more advantageous for more intricate correlated states, because once the coupling to the tailored environment is engineered, then you can just “set it and forget it.” The system naturally evolves to the desired state and stays there as long as the dissipation is happening.
Finally, we demonstrated that engineered dissipation has applications to non-equilibrium quantum phenomena beyond state preparation. Here we use a 1D system of 26 qubits to emulate a Heisenberg spin chain, a paradigmatic correlated system. We drive a current through the chain by periodically resetting the spins of two auxiliary qubits at either end. We first studied transferred magnetization, whose time dependence is observed to vary strongly depending on the underlying parameters of the model, in agreement with established theory (see below). At the so-called “isotropic point,” where exact solutions of the model are unknown, the team discovered a previously unknown phenomenon. At this point, we observed that the spin current has a distinct power-law dependence with time, which indicates a form of previously unknown, anomalous diffusion for this spin model. This result highlights the increasing functionality of the quantum processor as a tool for fundamental physics discovery, where the introduction of engineered dissipation unveils quantum phenomena that have never been observed or theoretically realized.
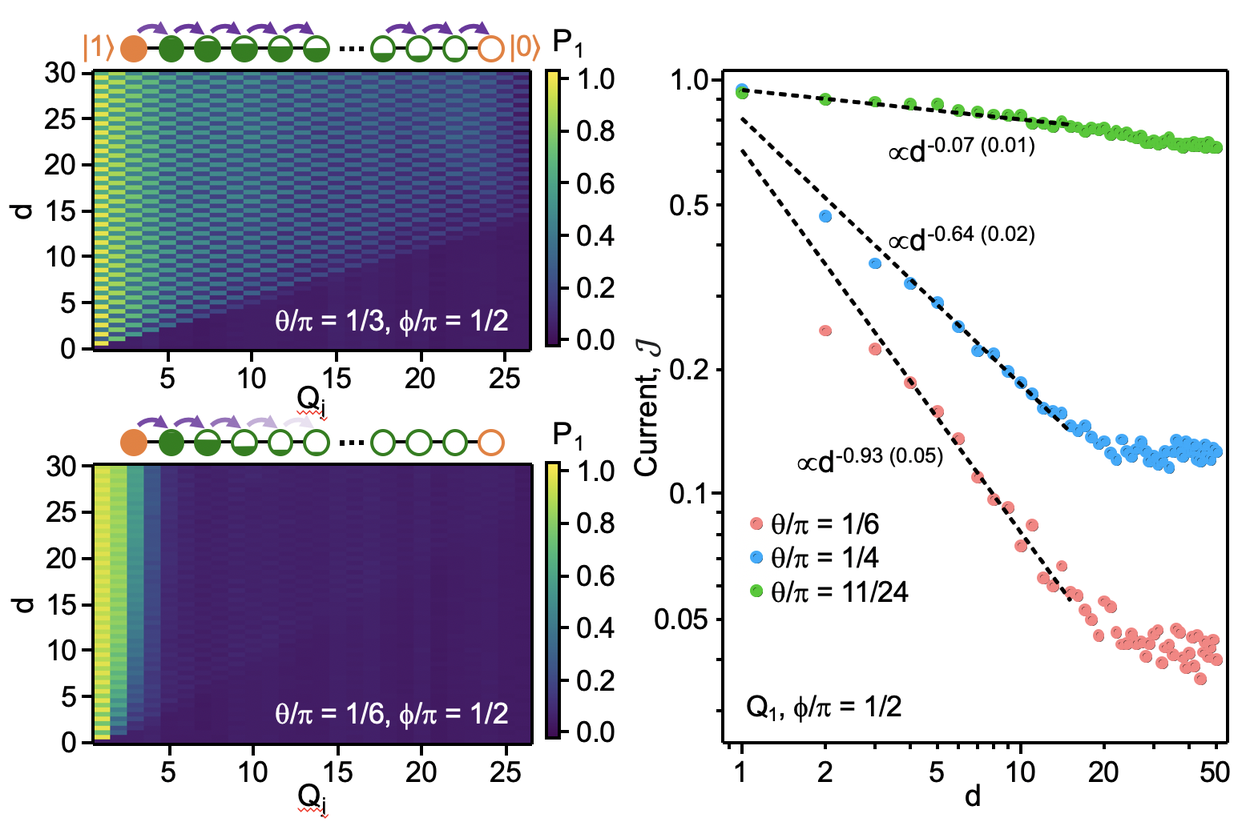
Left: Qubit polarizations as a function of time (vertical axis) and space (horizontal axis). Depending on the underlying parameters of the Heisenberg chain, the spin transport can be either ballistic (top panel) where the excitations move through very quickly, or diffusive (bottom panel) where the excitations move through very slowly. Right: Spin current as a function of time for different regimes of the Heisenberg chain. At the isotropic point (blue), we observe a previously unknown form of transport, called subdiffusive transport, in this well-known quantum magnetic model.
Conclusion
This work shows that engineered dissipation strongly enhances the functionality of quantum processors, allowing for a conceptually new approach to preparing and probing quantum-correlated states. Engineered dissipation will aid in the preparation of strongly correlated states, which give rise to new quantum phases of matter that are found in materials such as magnets. Quantum materials are considered among the most promising applications of quantum processors, yet most other protocols for correlated state preparation are severely limited by unwanted decoherence. We believe the methods demonstrated here will also enable the preparation of intricate many-body states such as quantum spin liquids, which may aid in the synthesis of high temperature superconductors or new materials for data-storage.
-
Labels:
- Quantum