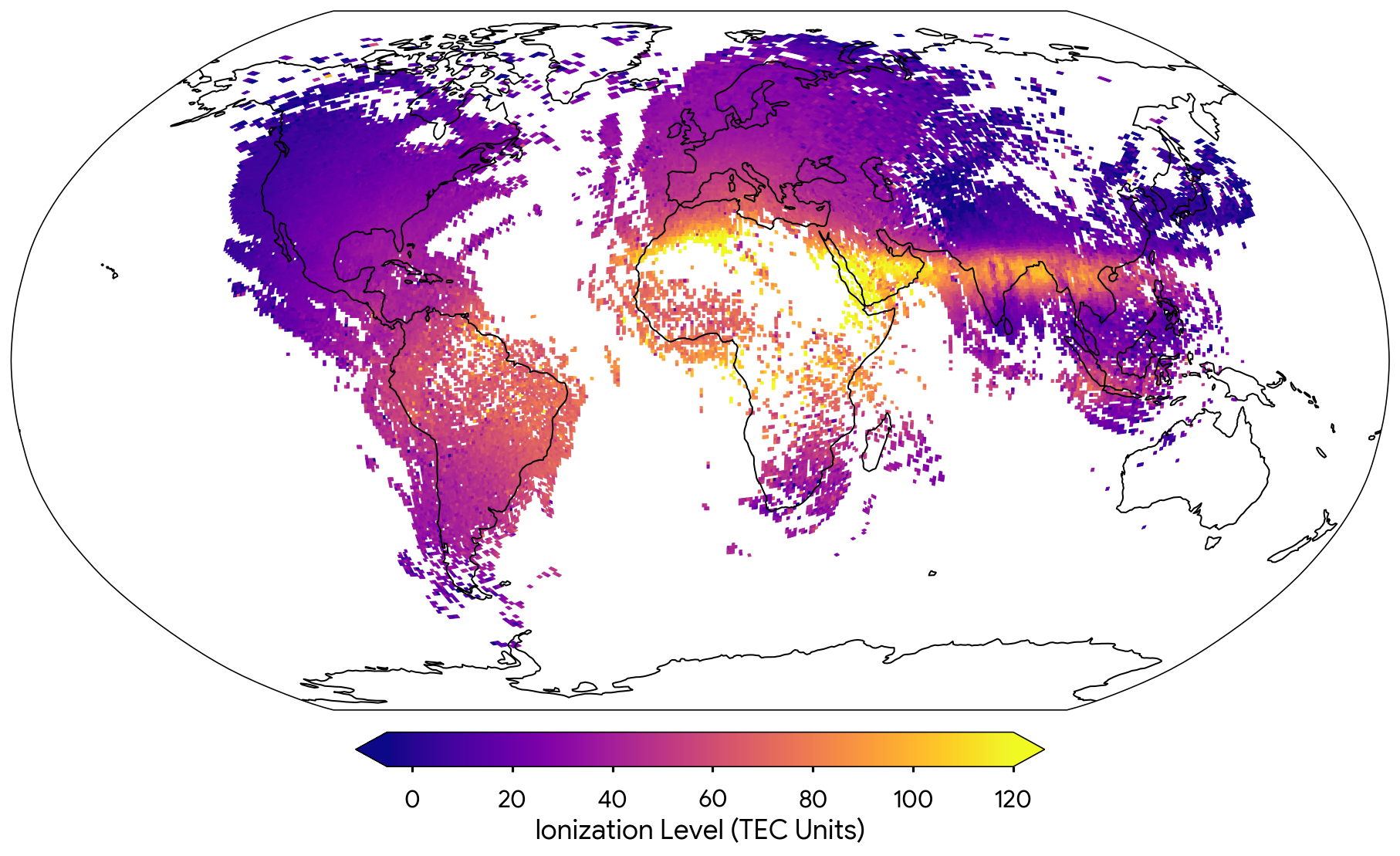
Mapping the ionosphere with the power of Android
November 13, 2024
Anton Kast and Jamie Smith, Staff Software Engineers, Google Research
We can now understand the ionosphere better thanks to aggregated measurements from millions of Android phones.
Quick links
The ionosphere is a swirling sea of charged particles that lies in Earth’s upper atmosphere. The ions accumulate when solar radiation collides with atmospheric gas molecules, knocking off some of their electrons. Variations in the free electron density across the ionosphere are one of the largest sources of error in satellite navigation systems such as the Global Positioning System (GPS). Spatial and temporal fluctuations in the ionosphere’s plasma based on the season, time of day, and geographical factors, such as distance from the equator, affect the trajectory of satellite signals and interfere with GPS location accuracy. More powerful disruptions to satellite communication and navigation systems are caused by bursts of radiation from solar storms — like the ones in May 2024, which produced spectacular aurorae across both the northern and southern hemispheres.
In a new study published today in Nature, we report the use of aggregated sensor measurements from millions of Android phones to map the ionosphere at a level of accuracy that matches — or, in some parts of the world, far exceeds — that of conventional monitoring infrastructure. Although each mobile phone on its own provides noisy readings, we demonstrate that a vast crowdsourced network of aggregated signals can act as a highly sensitive scientific instrument. Most importantly, we can accomplish this while maintaining important privacy protections — without identifying any contributing individual devices. This approach yields particular benefits in regions including India, Southeast Asia, and central Africa, where monitoring stations are sparse but mobile phone use is widespread.
Efforts are underway to deliver the location accuracy improvements enabled by this research to Android users. Knowing the current ionospheric conditions allows a GPS receiver to reduce location error by several meters. This level of increased accuracy could be incredibly helpful to users. For example, being able to differentiate between a highway and a parallel rugged frontage road can ensure dispatchers send the correct first responders to the correct place and provide help more quickly. Additionally, we found that mobile phone measurements capture scientifically important features of the ionosphere, providing unprecedented detail in regions with few monitoring stations. As worldwide concern grows about the risks and consequences of a major solar storm, phone sensor ionosphere mapping may provide needed insight into ionospheric phenomena.
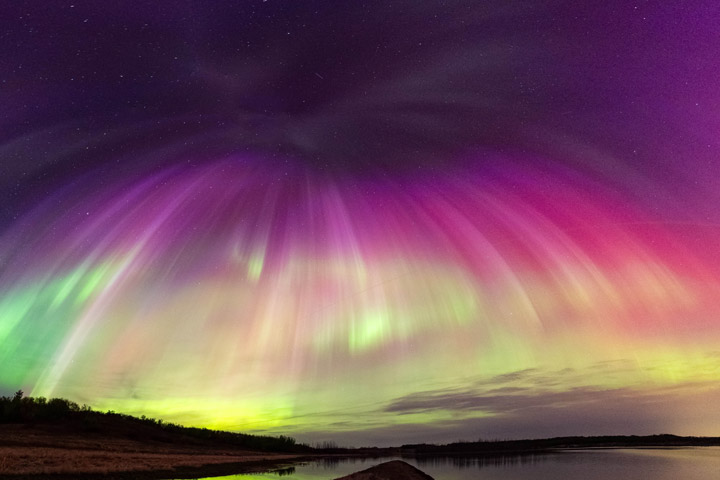
The stunning light displays of the aurora, such as this one observed on May 11, 2024, in Saskatchewan, Canada, occur when bursts of energy produced by solar storms interact with the earth’s magnetic field. Photo by Gunjan Sinha courtesy of NASA's Aurorasaurus project.
How the ionosphere affects GPS signals
GPS and other navigation signals originate from satellites orbiting about 19,000-40,000 km above the earth. These signals largely travel undisturbed through space until they hit the plasma of the ionosphere. Just as walking down a crowded street can slow you down, higher free electron concentrations in the ionosphere slow and disperse satellite signals. A GPS receiver relies on extremely precise timing of the radio signal to work out the receiver position. So, the delays caused by the ionosphere lead to location errors.
GPS receivers in most phones use a simple correction model that can remove about half of this error. We wondered whether advances in sensor technology available in today’s mobile phones, along with the large number of mobile phone users, could enable more detailed ionosphere mapping that could improve location accuracy.
Dedicated monitoring stations around the world detect satellite signals and correct for ionosphere-induced errors by measuring time differences between radio signals. Crucially, the delay between signals depends on the frequency of the radio signal as well as ionosphere conditions. So, if we measure the difference in arrival times for two signals from the same satellite on two different frequencies then we get a measurement of the ionosphere conditions along that path.
Mapping the ionosphere using dual-frequency Android phones
Many Android phones today have GPS or other receivers that can detect radio signals on two frequencies, so in principle, these mobile phones can accomplish the same calculation as stationary monitoring stations. But mobile phones have much smaller antennas, and unlike monitoring stations, which sit under an open sky, signals from some satellites are obstructed by buildings or even the person carrying the phone. Additionally, each phone has its own individual bias, adding a fixed offset on the order of microseconds to all its timing measurements, while the delay measurement is on the order of nanoseconds.
Satellite navigation signals pass through the ionosphere before arriving at phones on the ground. Ionization delays the signals by a few extra nanoseconds, and lower frequency signals (L5) are delayed a little more than higher frequency ones (L1). By measuring differences in arrival time, we can infer how much ionization there was along the signal path.
These biases must be measured and corrected to get meaningful results. Measuring with many phones solves this problem because we can figure out each phone's bias by comparing it to other phones. Two phones that receive signals passing through the same patch of sky at the same time should observe the same ionospheric delay. This constraint lets us infer how much bias each phone contributes by solving a gigantic consistency condition. Combining these biases with the differences in arrival times, we can map the ionosphere worldwide.
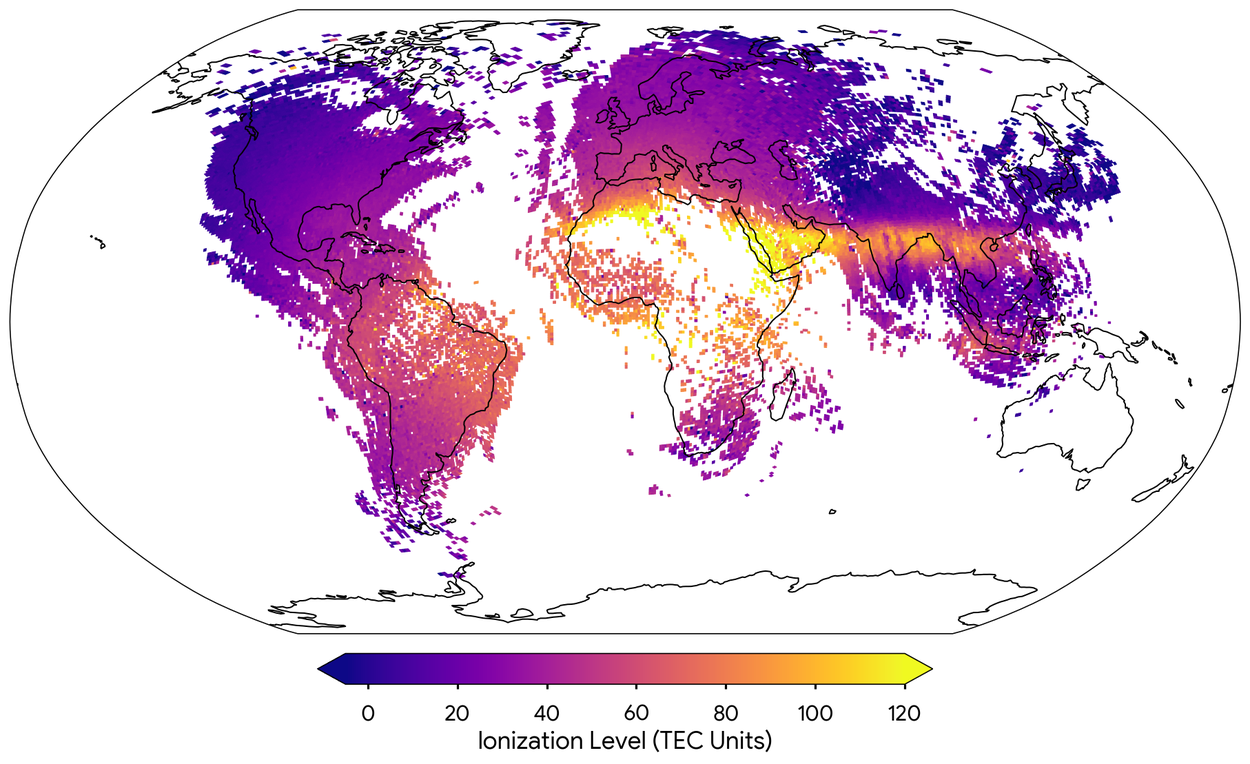
A worldwide map of atmospheric ionization made from ten minutes of phone measurements on October 12, 2023 at 2:00 p.m. UTC. The color gradient shows the amount of ionization, from purple (low ion density) to yellow (high ion density). The yellow horizontal stripe over south Asia is part of the equatorial anomaly. Data was captured in places where we had sufficient measurements: the daytime side of the earth and areas with greater population density.
Previous efforts to use mobile phone sensors to map the ionosphere were stymied by the fact that they were modeling measurements from just tens or hundreds of phones. The signal captured by each phone is so noisy that there has been significant skepticism in the field that ionosphere measurements from phones could produce usable data.
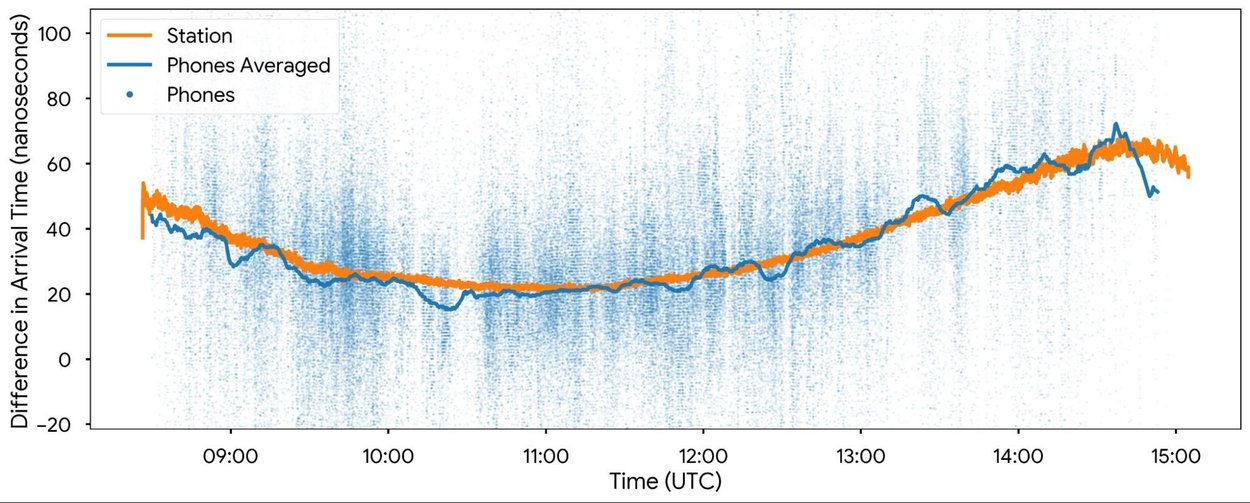
Each blue dot is a measurement of the ionization along the path from a satellite to the ground taken by phones in a particular city as the satellite passes overhead. The dots are widely dispersed, showing that each phone's measurements are imprecise. But phone measurements achieve accuracy when averaged (blue line). The averaged measurements follow the same curve as measurements from a monitoring station in the same city over the same time period (orange line).
For this proof of concept study, we used a set of mobile phone measurements captured during the daytime from the American GPS and European Galileo satellite constellations on the L1 and L5 frequencies — the most common dual frequency measurements used by mobile phones. These measurements included data from between 200,000 to 2 million phones per hour, with a total of about 40 million each day.
To combine measurements, we had to group them in space and time. This presented a tradeoff: smaller groupings give higher resolution, but bigger groupings yield better statistics. We settled on an ionosphere map grid of about 70 km and time intervals of 10 minutes as a balance between the two.
Privacy & data protection
Our ionosphere maps are created using aggregated measurements of the radio signals between satellites and the receivers in Android devices, and these measurements do not identify any contributing individual devices. These measurements come from a population of Android devices that already have location and relevant settings enabled and that are using satellite signals to determine location. You can read more about how Android uses this data in our privacy policy.
Each part of the ionosphere is measured by phones spread across a vast area, since the same part of the ionosphere can be measured from many locations using different satellites. Only the coarse location of a phone (within ~10 km) is used, and isolated phones in locations with low population density or with few other phones contributing measurements are excluded. Sensor calibration makes measurements from different devices more similar, and outlier measurements are removed. These steps help us understand the ionosphere better while obscuring the contribution from any individual device.
Increasing map coverage to capture distinct ionosphere features
Using a model that fit thousands of ionospheric parameters and millions of bias terms to the combined sensor measurements from millions of phones, we created detailed maps of free electrons in the ionosphere. We then compared measurements from our phone-based ionospheric maps to measurements from the Madrigal database, which contains data from about 9,000 monitoring stations.
Monitoring stations are prevalent across the US, Europe and other regions, such as Japan, Australia, New Zealand, South Africa, and parts of South America, but are sparse elsewhere. Ionospheric maps from these more sparsely covered regions have lower spatiotemporal resolution. Currently, these gaps are filled in by extrapolating from measurements taken earlier in the earth’s 24-hour rotation, as well as modeling based on historic averages of ionosphere dynamics. Our Android phone-based ionospheric maps cover twice as much of the ionosphere as do monitoring stations. We were thus able to significantly improve resolution in ionosphere maps from regions with few monitoring stations — in particular, Eastern Europe, South and Southeast Asia, much of Africa, and other parts of South America.
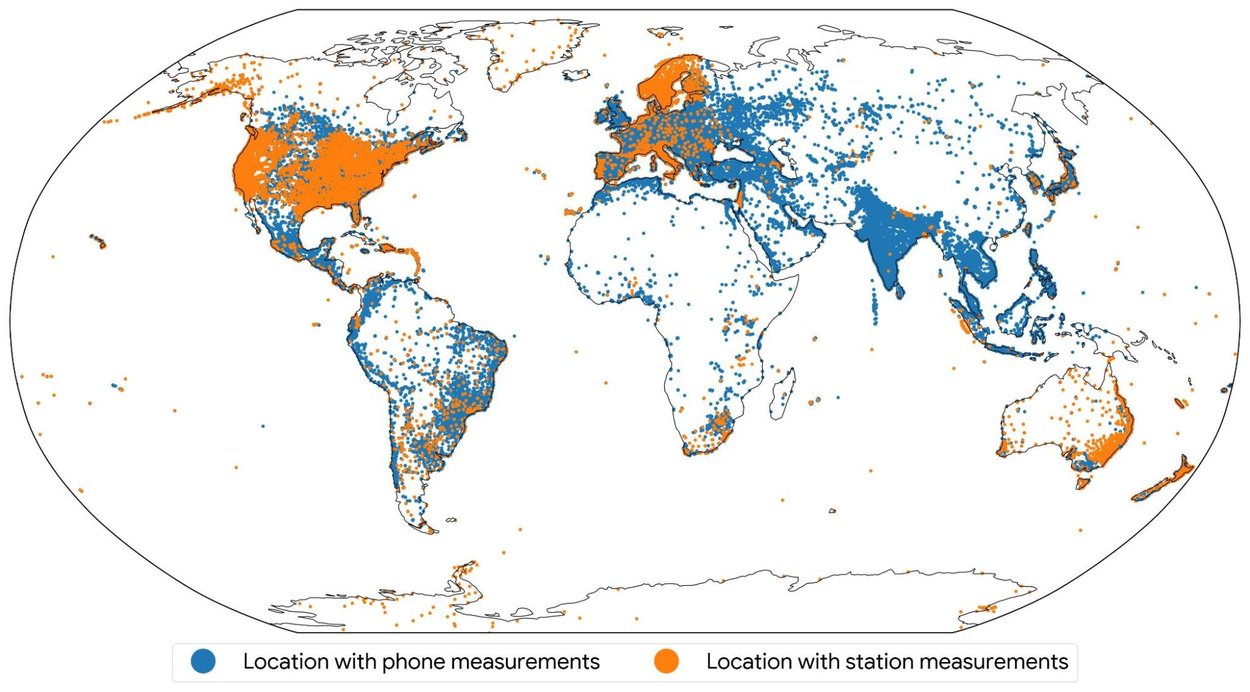
Worldwide coverage of the ionosphere by monitoring stations and by phones. Blue dots show about 100,000 locations where sufficient numbers of phone measurements are available. Orange dots show about 9,000 monitoring stations. Orange dots overlay blue dots. In blue areas, phones provide ionosphere coverage that was unavailable with monitoring stations alone.
Our results also show how phone-based ionosphere maps can lead to improved location accuracy for Android users, significantly outperforming the simple error correction model used on phones today. In many parts of the world, the performance of our model is equivalent to that of a state-of-the-art global ionosphere map created using measurements from monitoring stations. However, in places like India, which has dense mobile phone coverage but few monitoring stations, our model provides performance that is even better than maps generated from monitoring station measurements.
Our phone-based maps provide a clear view of ionospheric fluctuations and disturbances. For example, our data capture the characteristic crests and dips of a phenomenon called the ionospheric equatorial anomaly. They also show the signature of a major solar storm that took place on May 10-11, 2024 in the form of increased ionospheric activity over North America. Some of the phenomena we captured have not been previously registered at this level of detail by measurements from monitoring stations. For example, we observed eastward-moving waves of reduced ion density over South Asia and Brazil corresponding to structures termed plasma bubbles, which are known to disrupt GPS signals.
The advantage provided by phone measurements (right) over monitoring stations (left) is most striking in parts of the world like South Asia where there are few monitoring stations but many phones. The purple waves moving eastward across India are pockets of lower ionization called "plasma bubbles", which can interfere with radio communication as well as satellite navigation. The animation covers the interval from 13:00 to 16:00 UTC on October 14, 2023.
The Americas on the day of a solar storm (left, May 10, 2024) and on a calm day a week prior to that (right) as seen by Android phones from 18:00 to midnight UTC. The bright yellow on the left shows the exceptional degree of ionization over the U.S. during this unusual event.
Benefits of phone-based ionosphere maps
We demonstrated how a vast network of phones could act as a powerful scientific instrument, enabling measurement of a global natural phenomenon. This will in turn translate into better GPS location accuracy for Android users.
Improving the coverage and resolution of global ionosphere mapping also has important societal and scientific implications. Geomagnetic events in the ionosphere, such as those produced by solar storms, have the capacity to disrupt power grids, radio communications and navigation systems, thereby upsetting key elements of societal infrastructure, including emergency response operations, healthcare and government. Although solar storm preparedness is an urgent priority nationally and internationally, scientists have a limited understanding of space weather prediction. Our research offers new insight into ionosphere dynamics during solar storms and may help support new discoveries in ionosphere science.
Our efforts align with other projects that aim to use mobile phone sensors to measure natural phenomena. Scientists have demonstrated, for example, that measurements from phone barometers can aid in weather forecasting and that the accelerometers on phones can detect earthquakes to act as early warning systems. We are excited to further illustrate how the devices that so many of us carry in our pockets can be used to provide useful measurements, which in turn could benefit society and expand our scientific understanding of the planet.
Acknowledgements
We would like to acknowledge the many contributors to the paper, especially the other members of the core research team (Anton Geraschenko, Jade Morton, and Brian Williams) and our colleagues in Android (Frank van Diggelen, Imad Fattouch, Ari Moradi, Jennifer Wang, Jordan McClead and Emily Liu). Thanks also to Alla Katsnelson and John Guilyard for assistance with this blog post, and to Lizzie Dorfman, Michael Brenner, and John Platt for their support and leadership.