Accurately computing electronic properties of materials using eigenenergies
Alan Derk
Alan Ho
Alex Opremcak
Alexander Korotkov
Andre Gregory Petukhov
Andrew Dunsworth
Anthony Megrant
Bálint Pató
Benjamin Chiaro
Bob Benjamin Buckley
Brooks Riley Foxen
Charles Neill
Cody Jones
Daniel Eppens
Dave Landhuis
Doug Strain
Edward Farhi
Eric Ostby
Fedor Kostritsa
Frank Carlton Arute
Igor Aleiner
Jamie Yao
Jeremy Patterson Hilton
Jimmy Chen
Josh Mutus
Juan Atalaya
Juan Campero
Kostyantyn Kechedzhi
Kunal Arya
Marco Szalay
Masoud Mohseni
Matt Jacob-Mitos
Matt Trevithick
Michael Blythe Broughton
Michael Newman
Nicholas Bushnell
Nicholas Redd
Orion Martin
Pavel Laptev
Ping Yeh
Rami Barends
Roberto Collins
Sean Harrington
Sergei Isakov
Thomas E O'Brien
Trent Huang
Trevor Mccourt
Vadim Smelyanskiy
Vladimir Shvarts
William Courtney
William J. Huggins
Wojtek Mruczkiewicz
Xiao Mi
Yu Chen
arXiv preprint arXiv:2012.00921(2020)
Abstract
A promising approach to study quantum materials is to simulate them on an engineered quantum platform. However, achieving the accuracy needed to outperform classical methods has been an outstanding challenge. Here, using superconducting qubits, we provide an experimental blueprint for a programmable and accurate quantum matter simulator and demonstrate how to probe fundamental electronic properties. We illustrate the underlying method by reconstructing the single-particle band-structure of a one-dimensional wire. We demonstrate nearly complete mitigation of decoherence and readout errors and arrive at an accuracy in measuring energy eigenvalues of this wire with an error of ~0.01 radians, whereas typical energy scales are of order 1 radian. Insight into this unprecedented algorithm fidelity is gained by highlighting robust properties of a Fourier transform, including the ability to resolve eigenenergies with a statistical uncertainty of 1e-4 radians. Furthermore, we synthesize magnetic flux and disordered local potentials, two key tenets of a condensed-matter system. When sweeping the magnetic flux, we observe avoided level crossings in the spectrum, a detailed fingerprint of the spatial distribution of local disorder. Combining these methods, we reconstruct electronic properties of the eigenstates where we observe persistent currents and a strong suppression of conductance with added disorder. Our work describes an accurate method for quantum simulation and paves the way to study novel quantum materials with superconducting qubits.
Research Areas
Learn more about how we conduct our research
We maintain a portfolio of research projects, providing individuals and teams the freedom to emphasize specific types of work.
Our research philosophy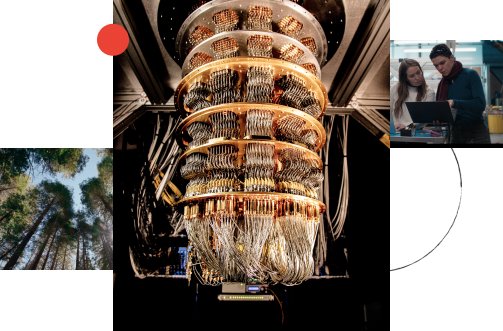